NIF success gives laser fusion energy a shot in the arm
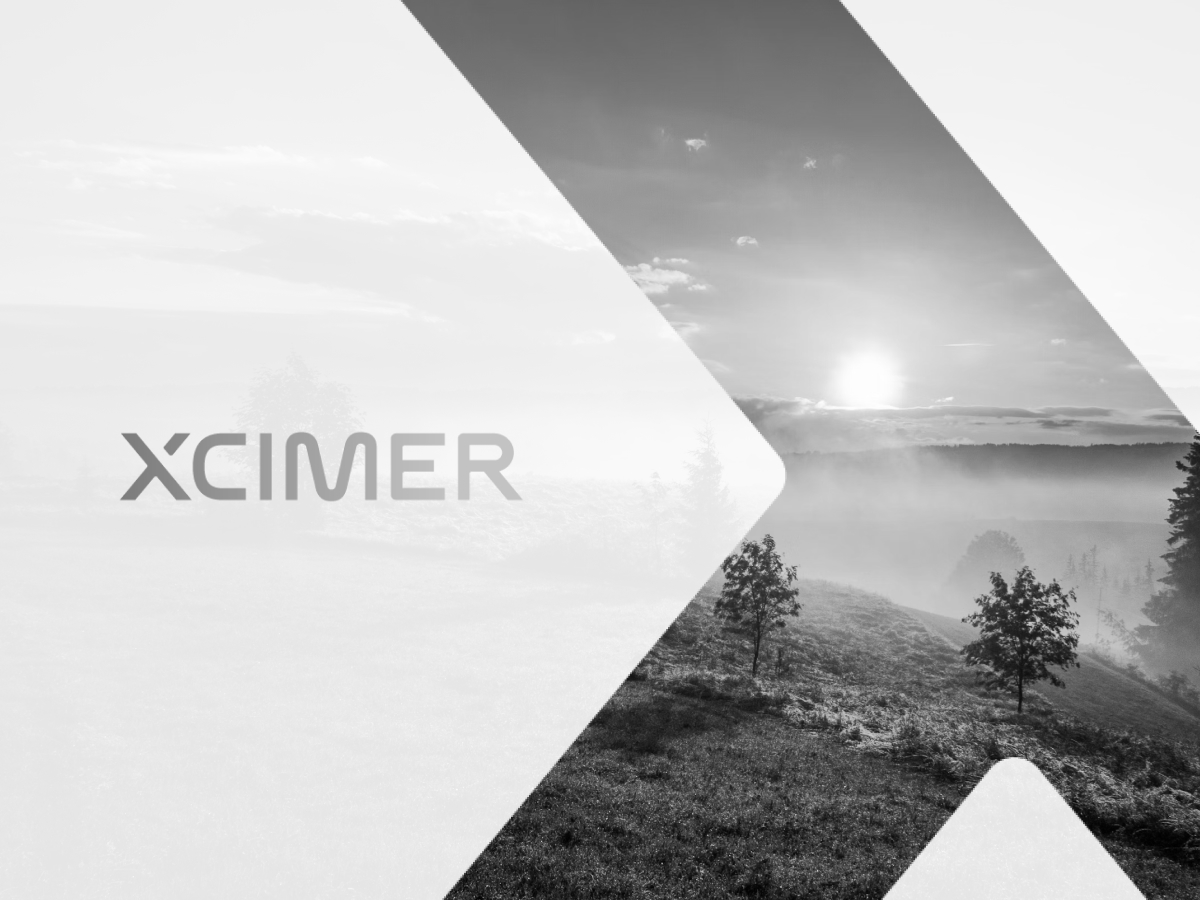
Startup companies are betting on different approaches and laser technologies for fusion to become a commercially viable energy source.
The attainment of fusion ignition and energy gain on the world’s most energetic laser late last year was indisputably a major scientific accomplishment. But the road to fusion as a viable source of energy will be a long one, if not a dead end. And if it does ultimately become a reality, most experts say that it is unlikely that a laser-driven fusion power plant will be based on the approach taken by the National Ignition Facility (NIF), where the fusion milestone occurred.
The December shot, which produced 1.5 times the 2 MJ of energy that was fired on the fusion fuel, has silenced skeptics who said that ignition could never be created by bombarding tiny capsules of deuterium–tritium fuel with lasers. (See “National Ignition Facility surpasses long-awaited fusion milestone,” Physics Today online, 13 December 2022.) “They have done something very important: demonstrating ignition and burn,” says Stephen Bodner, a retired head of the laser fusion branch at the US Naval Research Laboratory who once was a persistent critic of NIF’s approach.
And the milestone is likely to open the floodgates to new investments in the handful of startups that are pursuing inertial fusion energy (IFE). “I think you will see a proliferation of companies devoted to IFE or aspects of IFE because of this and because of investor interest,” says Todd Ditmire, a University of Texas at Austin physicist who is chief technology officer of Focused Energy, an IFE startup.
Yet despite the fanfare greeting the announcement, the fact is that the fusion energy yield from the successful shot amounted to less than 1% of the 300 MJ taken from the electricity grid to power NIF’s 192 beams. And the energy released was enough to boil about 10 tea kettles. Many experts say that economically viable fusion will require fusion reactions yielding energy gains of at least 100 times the energy deposited on the fuel capsule—two orders of magnitude greater than the NIF shot.
. A laser fusion power plant proposed by Longview Fusion Energy Systems would generate 1000 MWh or more of electricity. The plant would compress fusion fuel by using indirect drive, the same approach used at the National Ignition Facility, which in December announced that it had produced ignition and gain, the first time that fusion researchers have attained those milestones.
A laser fusion power plant proposed by Longview Fusion Energy Systems would generate 1000 MWh or more of electricity. The plant would compress fusion fuel by using indirect drive, the same approach used at the National Ignition Facility, which in December announced that it had produced ignition and gain, the first time that fusion researchers have attained those milestones.
Bedros Afeyan, a consultant who has worked in fusion R&D at three national laboratories, estimates that the NIF accomplishment places IFE at 10% of the way to commercialization. “IFE is so difficult that the solution will be a black swan,” he says. “Whatever the idea ends up being, it will be unique.”
An IFE power plant will need to fire a laser shot at least every few seconds, compared with the several-hours interval between NIF shots. The machine will also need to breed its own fuel and to load it into tiny capsules that somehow must be kept at cryogenic temperatures for a split second after they are injected into a hellishly hot reactor chamber. And the plant must cost-competitively produce either electricity, industrial process heat, or an energy storage medium such as hydrogen.
Direct or indirect?
At least three fundamental questions need to be resolved as IFE developers move on from NIF, which was designed not for energy production but to simulate processes that occur in nuclear weapons. First, will the laser’s light implode fuel capsules directly, or should NIF’s indirect-drive approach, where the light is first converted to x rays to squeeze the pellets, be emulated? Second, what type of laser can best do the job? And finally, what is a viable path to designing and mass-producing the targets containing the D–T fuel at minimal cost? The answers to those questions will be key to whether laser fusion can be made economical.
Two US startups—Focused Energy and LaserFusionX—are pursuing direct drive, using different laser types. Longview Fusion Energy Systems, based in Orinda, California, is developing a NIF-style, purely indirect-drive approach. Xcimer Energy, in Redwood City, California, has proposed a hybrid indirect–direct scheme.
Many laser experts say indirect drive can’t be made efficient enough to achieve the level of gain necessary to produce electricity at an acceptable cost. Michael Campbell, retired director of the Laboratory for Laser Energetics at the University of Rochester, says too much laser energy is lost during the absorption of UV rays and emission of x rays that occur inside the hollow cylinder, or hohlraum, that surrounds the capsule of fusion fuel.
The more complex targets required for indirect drive are likely to be more expensive than the simple spherical fuel capsules that are being proposed for direct drive. The NIF targets, which are not mass-produced, cost $10 000 or more apiece. To be viable, each of the hundreds of thousands of individual targets that will be imploded each day will have to cost less than $1. Target design must be kept as simple as possible, says Afeyan. “Forget about indirect drive. It’s out of the question,” he says.
The hohlraums of indirect drive could provide a modicum of protection for capsules containing cryogenic D–T fuel as they are injected in rapid-fire fashion into the target chamber. But debris from exploded hohlraums could rapidly pile up, potentially presenting a cleanup problem.
Direct drive has been pursued mostly at the Laboratory for Laser Energetics, which is supported by the Department of Energy and houses the Omega laser. Direct-drive researchers have so far been unable to produce implosions with the precise symmetry required for ignition. The more energetic the laser, the less precision will be required for direct-drive implosions that would produce ignition and gain, Campbell says.
Omega is too small in any case, Campbell says, producing just 25 KJ of light. “There needs to be another research facility built with enough energy to get plasmas to ignite in a direct-drive configuration.”
Glass versus gas
The two primary laser candidates today are the NIF-like solid-state glass system and the excimer, which uses krypton fluoride or argon fluoride gas. Excimer lasers, which pump and excite a gas with electron beams instead of photons, are more efficient, but for IFE use, they will require innovations in pulsed power and nonlinear optical elements that can amplify without glass or mirrors, Afeyan says.
LaserFusionX, based in Springfield, Virginia, and Xcimer are pursuing different types of excimer lasers. Focused Energy and Longview are using glass lasers.
Afeyan says the feasibility of IFE will hinge on whether lasers can be made sufficiently large—providing 20–30 MJ to the target. Simple, cheap targets can’t be driven using simple lasers, he says. “If the targets are cheap, the laser has to be huge.” That, he says, eliminates glass lasers from consideration. “Making a glass laser 100 or 50 times bigger [than NIF] is out of the question. Glass lasers are inherently inefficient.” Heat buildup will keep them from ever becoming efficient enough to operate a million or more times each day, no matter how well they are cooled, he adds.
. The target bay of the National Ignition Facility (NIF), where the world’s first laboratory-scale fusion ignition and energy-gain experiment occurred in December. NIF’s 192 beams converge at the center of a spherical target chamber covered with beamlines and diagnostic instruments and deposit just over 2 MJ of UV light onto a target containing a tiny sphere of deuterium–tritium fuel. The bay served as the set for the engine room in the 2013 movie Star Trek: Into Darkness.
The target bay of the National Ignition Facility (NIF), where the world’s first laboratory-scale fusion ignition and energy-gain experiment occurred in December. NIF’s 192 beams converge at the center of a spherical target chamber covered with beamlines and diagnostic instruments and deposit just over 2 MJ of UV light onto a target containing a tiny sphere of deuterium–tritium fuel. The bay served as the set for the engine room in the 2013 movie Star Trek: Into Darkness.
Ditmire insists that glass’s thermal problems can be managed. He notes that Lawrence Livermore National Laboratory (LLNL) has built 100 J, 10 Hz glass lasers that use helium cooling. And the Pentagon has done considerable work on thermal management in glass-laser directed-energy weapons, he says.
“From a physics standpoint, excimers have a problem in that they don’t store energy,” says Ditmire. Glass stores energy in the upper laser state for hundreds of microseconds, thus allowing for the extraction of the nanoseconds-long pulses needed for implosions, he says. “The amount of energy per square centimeter that I can get out of a glass laser is much higher than in an excimer laser.”
Glass lasers will need to fire multiple times each second. An LLNL program known as Laser Inertial Fusion Energy (LIFE; see Physics Today, April 2014, page 26) explored what an IFE power plant with indirect drive might look like. Discontinued in 2014, LIFE estimated a rate of 16 Hz—using 1.3 million targets per day.
Focused Energy’s approach calls for two solid-state lasers producing pulses for each shot: a nanoseconds-long pulse to implode the target and a picoseconds-long pulse to then ignite the fuel. Known as fast ignition, the technique differs from the “hot spot” implosion of NIF, which, like a diesel engine, relies on compression alone. Focused Energy aims to use 180 beams—80 to implode the capsule and 100 for the fast-ignition spark plug, Ditmire says.
The NIF results have stimulated interest from investors, Ditmire says. “The timing couldn’t have been better. We are seeing investors coming out of the woodwork.” Focused Energy is currently closing a $60 million funding round, and it plans to raise another $200 million to build a single-beam prototype laser in Austin by 2027. That would be followed by a second laser in Texas that the company hopes will achieve ignition and gain by the early 2030s. A third demonstration laser also is planned that would fire at 10 Hz.
Marvel Fusion in Germany and Australia’s HB11 Energy also propose fast ignition, but they seek to combine boron and protons rather than D–T. The p–11B reaction, although offering the environmental benefit of producing virtually no neutrons, requires temperatures of 3 billion kelvin, about 10 times what is needed to burn D–T. (See “The commercial drive for laser fusion power,” Physics Today online, 20 October 2021.)
Xcimer’s indirect–direct hybrid approach offers the thermal shield provided by a hohlraum, which also confers “significant smoothing of the laser imprint” on the fuel capsule, says CEO Conner Galloway. Only a small fraction of the laser’s energy will be directed at the hohlraum; most of the energy will be used for pulses from the same laser that are deposited directly on the fuel. Xcimer’s scheme borrows from the 1980s-era Strategic Defense Initiative, which used high-power KrF lasers and stimulated Brillouin scattering, a nonlinear optical method for compressing the laser’s microseconds-long pulses to the nanoseconds needed for implosions. Because of anticipated energy gains of up to 150, Galloway says, a 10 MJ KrF laser driver would need to fire only once per second, or even every few seconds, to produce grid-scale energy.
Excimer lasers can be much less costly than glass, Galloway says. He explains that NIF’s $3.5 billion cost included 120 tons of expensive glass-laser amplifier slabs and a total optical area of 30 m2. Xcimer’s use of a gas-amplifying medium and a beam that’s manipulated with “gas mirrors” instead of glass makes the approach cheaper.
In the future, Xcimer may be able to dispense with the hohlraum, which would moderately increase the fusion gain of the target, Galloway says. As with other fusion startups, the company plans to pursue additional revenue opportunities for the laser technologies it develops on the way to its IFE goal.
Xcimer has raised $12 million to date from several venture capital firms, including Lowercarbon Capital, Prima Ventures, Starlight Ventures, and Wireframe Ventures. Another funding round, expected to be completed this spring, will finance a multi-kilojoule laser to be completed in two years. That device will prove out the fundamental concept and scalability of using the pulse compression technique, Galloway says. That is slated to be followed by a 4 MJ prototype in 2028 that could achieve target gains of 30–50. He anticipates a working fusion energy pilot plant with a 10 MJ laser that produces grid-scale power in 10 years.
With the incorporation of LaserFusionX last year, Stephen Obenschain is hoping to commercialize ArF excimer laser technology developed at the Naval Research Laboratory, from which he retired last year. He says that ArF produces shorter-wavelength light with a broader bandwidth than KrF and should therefore better suppress instabilities in the fusion plasma. A commercial-scale pilot plant could be built in 16 years, he says.
Obenschain has been financing his effort from his personal investments. He’s talking to venture capitalists, and he hopes to get some help in those discussions from DOE’s Advanced Research Projects Agency–Energy. “The challenge you run into is if you ask for too much, the billion-dollar VCs won’t do it, and if you ask for too little, it’s not exciting. I’m trying to find the middle ground,” he says.
Longview CEO Ed Moses, a former NIF director, points out that indirect drive is the only approach that has demonstrated ignition and gain. Borrowing from the LIFE program, Longview plans to achieve gains of 50–60 from targets imploded at a rate of 15 Hz. The concept would require producing more than a million targets per day, or 500 million a year. That’s not only feasible, Moses says, but it is also less difficult than manufacturing bullets for the military. Each target, costing 50¢ or less, would consist of a fuel capsule surrounded by a hohlraum made of lead. Several utilities have shown interest in his proposal to build gigawatt-scale power plants costing about $4.5 billion, which he says is typical for other types of baseload generating stations. The plants could be sited at retiring fossil-fuel-fired facilities to make use of their existing turbine and transmission infrastructure, he says.
Longview plans on using diodes to pump its 384 laser beams. Unlike NIF’s flashlamps, which produce white light, just 3% of which is absorbed by the laser optics, diodes can be tuned to the laser’s absorption frequency. That makes the transfer of light to the laser close to 100% efficient, Moses says. Plans call for five years of design work and reducing technology risks, to be followed by the five-year-long construction of the first laser prototype. To minimize thermal problems, Longview will use optical elements that are one-fifth as thick as NIF’s glass. The tons of lead per month that would accumulate from the exploding hohlraums can be recycled into new targets, which would be fabricated on-site, Moses says. He declined to discuss the company’s financing, investors, or the size of its workforce.
Other technology needs
To be sure, many other challenges need to be dealt with before IFE becomes a reality. Some are common to both IFE and tokamak and other magnetic fusion schemes. Those include how to breed required amounts of tritium, purify it, and load it into targets. Another is finding materials that can protect reactor walls from damage caused by the high-energy neutrons and x rays that will be constantly bombarding them. Many developers are counting on FLiBe, a molten salt made from a mixture of lithium fluoride and beryllium fluoride. Some propose using it as a blanket to line the chamber. Others would locate the material behind a solid wall made of tungsten or other radiation-resistant metal. In either case, high-energy neutrons would initiate a nuclear reaction that transmutes the lithium in the salt to tritium.
A report from a DOE Fusion Energy Sciences workshop held last year on basic research needs for IFE says that a suite of facilities will be needed “to increase the rate of learning and test new technologies.” Those facilities range from “‘at scale’ physics facilit(ies) for testing concepts to a wide range of component and sub-system development facilities.” Responding to congressional urging, DOE’s fusion program established a $3 million IFE effort in the current fiscal year.
1. D. Kramer, “National Ignition Facility surpasses long-awaited fusion milestone,” Phys. Today, 13 December 2022 . https://doi.org/10.1063/PT.6.2.20221213aGoogle Scholar
2. D. Kramer, Physics Today 67(4), 26 (2014). https://doi.org/10.1063/PT.3.2344Google ScholarCrossref
3. D. Kramer, “The commercial drive for laser fusion power,” Phys. Today, 20 October 2021 . https://doi.org/10.1063/PT.6.2.20211020aGoogle Scholar
© 2023 American Institute of Physics.